Three new EAM Starting Grants 2023/2024 awarded
There was a high level of competition for this year’s EAM Starting Grant. In the end, the Executive Board of the FAU Competence Center EAM decided to award three new Starting Grants to FAU researchers. The EAM Starting Grant aims to support young researchers to pursue their own research projects and thus gaining a boost for a successful academic career. The grant is intended to bring new contributions and ideas into FAU EAM’s research activities and serves as seed funds for the preparation of new proposals. For 2023-24 the following awardees were chosen:
Kinetic Pathways of Lower Symmetry Nanocrystal Habits
Dr. Carlos Lange Bassani, 32
Humboldt Postdoctoral Fellow at the Institute for Multiscale Simulation
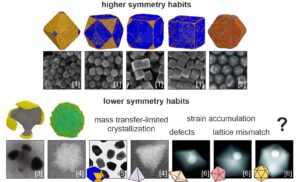
The possibility of assembling nanocrystals (NCs) into superlattices represents a new way of tuning material properties. The variety of NC habits and the possibility of their surface functionalization with shorter or longer-range interactions makes it possible to trespass the barriers so far believed in chemistry. Experimentalists thrived in forming NCs with controlled size and shape and assembling them into different superstructures. Their functionality nevertheless depends on the precise control of NC habits and superstructure formation, as well as on the electronic coupling between NCs – that is, an inherently multiscale process -, but multiscale models nevertheless did not keep the pace of the recent advances in the field. This project tackles the upscaling of atomic to realistic-sized NC scales formed of 10s-of-millions atoms via a new rejection-free Kinetic Monte Carlo method based on the semi-Gibbs ensemble. Of special attention is the role of strain accumulation via defects, lattice mismatch, and geometric frustration in changing the dynamic pathway of crystalline growth, thus kinetically entrapping NCs into lower symmetry habits – that is, shapes that do not comply with the underlying symmetry of the crystalline structure. The coupling with reactor scales (environment) to understand mass transfer-limited crystallization is also pivotal to predicting the yield of denser NC populations. Bridging the multiscale understanding from atom to NC to the environment will make it possible to optimize NC synthesis conditions and potentially lead to discovering new NC habits.
References of microscope image: [1] Xia et al, J. Am. Chem. Soc. 2012, 134 (3), 1793–1801, [2] Ahn et al, J. Mat. Chem. C 2013, 1, 6861, [3] Chen et al, Nature Comm. 2020, 11 (1), 3041, [4] Sun et al, ACS Nano 2021, 15 (10), 15953–15961, [5] Xia and Xia, Nano Lett. 2012, 12 (11), 6038–6042, [6] Langille et al, Science 2012, 337 (6097), 954–957.
Probing Electrochemical Atomic Layer Deposition
Prof. Dr. Siow Woon Ng, 35
Junior Professor for Physical Chemistry at Interfaces
Atomic layer deposition (ALD) remains as a leading thin film deposition technique. Its signature characteristics of self-limiting surface reactions offers exceptionally well controlled growth: Atomic precision of layer thickness, high-quality homogeneous films, and conformal coverage. ALD is highly favored for preparing functional, seeding or passivation layers, for a wide range of materials in micro- and opto-electronics, photovoltaics, catalysis, batteries, sensors, and bio-medical industries. Electrochemical atomic layer deposition (E-ALD) combines the strength of two liquid phase deposition techniques: electrodeposition and solution ALD. E-ALD explores electrochemical control of chemical interactions with the substrate in liquid environment, while preserving the self-limiting surface reaction. This approach broadens the options of precursor and allows the deposition at more reasonable conditions compared to conventional ALD. This project aims to investigate electrochemical surface-limited reactions, observe the nucleation and growth of thin films using scanning probe electrochemical methods, and ultimately striving for atomic-level control of deposition.
SPACE – Superior Performance through Accelerated Composition Exploration
Dr.-Ing. Christopher H. Zenk, 37
Group Leader High Performance Alloys
Power generation and propulsion demand cutting-edge alloys operating in extreme conditions. Superalloys, key to efficient gas turbines and aerospace engines, not only require an excellent high-temperature creep strength, but also exceptional resistance to oxidation and corrosion. However, their significance expands beyond current technologies, as novel high-performance alloy systems are also key in clean concepts like hydrogen-fueled aircraft engines and high-temperature solid-state oxide fuel cells. Additionally, in concentrated solar power plants and nuclear fission reactors, steam turbines are the key component to convert the generated heat into usable electricity. Even in future nuclear fusion reactors, steam turbines are envisaged to extract the massive thermal energy of the plasma and its efficiency increases with the operating temperature, which in turn is limited by the materials’ high temperature strength in presence of steam.
SPACE – “Superior Performance through Accelerated Composition Exploration” aims to revolutionize alloy discovery, development, and characterization. Unlike traditional, time-consuming methods, SPACE employs a combinatorial approach, producing and testing compositionally graded bulk specimens. Through Laser-Based
Directed Energy Deposition (L-DED) in inert Ar-atmosphere and high-throughput characterization, SPACE will assess phase stability, microstructure, diffusion, corrosion resistance, and mechanical properties on the meso-scale as a function of composition, atmosphere, temperature, and time.
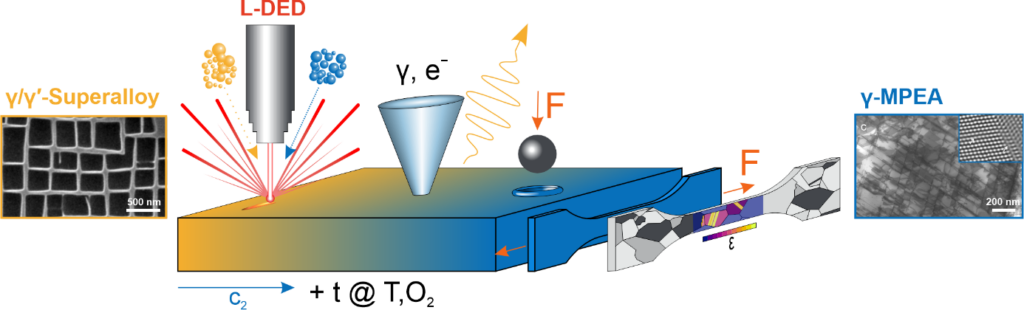